Biological computing, an emerging interdisciplinary field at the intersection of biology and computer science, is poised to revolutionize the pharmaceutical industry. At its core, biological computing leverages biological organisms or processes—such as DNA, molecules, and cells—to perform computational tasks traditionally handled by electronic computers. This innovative approach encompasses key technologies like DNA computing, where DNA molecules are used for data storage and processing; molecular computing, which employs chemical reactions to perform computations; and cellular computing, which utilizes the natural functions of living cells to process information.
The journey of biological computing from theoretical concepts to practical applications began in the mid-20th century. The pioneering work of Leonard Adleman in the 1970s marked a significant milestone when he demonstrated the potential of DNA strands to solve complex mathematical problems, such as the Traveling Salesman Problem. This breakthrough not only showcased the computational capabilities of biological systems but also laid the groundwork for subsequent advancements in the field. The development of genetic logic gates and synthetic biology further propelled biological computing into new realms of possibility, enabling the creation of complex biological circuits that mimic electronic systems.
One of the most notable achievements in biological computing is the successful application of DNA computing to solve the Traveling Salesman Problem—a classic optimization problem in mathematics and computer science. By harnessing the unique properties of DNA, researchers have demonstrated the potential of biological systems to handle tasks that are computationally intensive for traditional computers. Moreover, the advent of synthetic biology has enabled scientists to engineer biological circuits with precision, paving the way for innovations like biosensors for environmental monitoring and targeted drug delivery systems in medicine.
As biological computing continues to evolve, its implications for the pharmaceutical industry are profound. The ability to process vast amounts of biological data quickly and accurately can accelerate drug discovery, personalize medical treatments, and improve the efficiency of clinical trials. With companies like Cortical Labs exploring the integration of brain cells with computer chips, the future of bio-computer hybrids holds promise for even greater advancements in healthcare and beyond.
Bio-Computer Hybrids: The Future of Computing
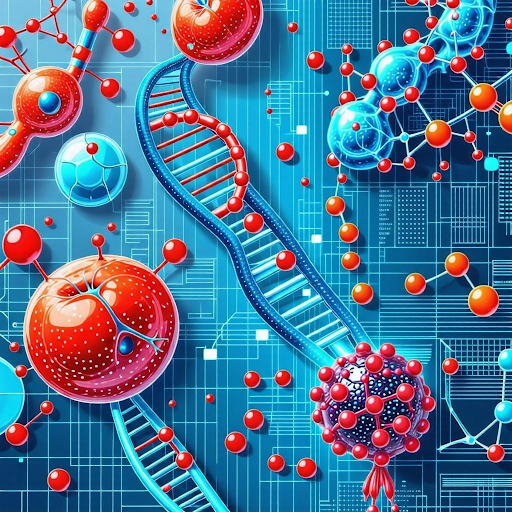
As biological computing capabilities continue to advance, the integration of biological and conventional electronic components is emerging as a promising new paradigm known as bio-computer hybrid systems. These innovative platforms seek to merge the strengths of living and non-living matter by combining biological molecules, cells, and tissues with traditional silicon-based circuitry.
One of the most notable examples of this innovation comes from Cortical Labs, which is pioneering the development of bio-computer hybrids by growing brain cells on computer chips. This approach, exemplified by their “DishBrain” project, seeks to harness the natural computational capabilities of neurons to perform complex tasks that are challenging for conventional silicon-based systems.
Energy Efficiency and Sustainability
A major motivation for developing bio-computer hybrids is their potential energy efficiency gains over conventional computing. While electronic devices consume large amounts of energy for tasks like data transmission and processing, biological systems accomplish the same functions using surprisingly little power.
For example, the human brain operates at around 20 Watts despite containing nearly 100 billion neurons and quadrillions of synapses.
Researchers estimate that bio-hybrid systems could achieve 1000x increases in energy efficiency relative to today’s most advanced supercomputers. This would address rising concerns about the sustainability of power-hungry data centers run by tech giants like Amazon and Microsoft. Just a modest 1% improvement in server farm performance could save over $1 million annually in electricity costs alone.
Applications in Pharma
DishBrain, developed by Cortical Labs, exemplifies the potential applications in this field. This bio-computer hybrid can simulate complex biological processes, offering a powerful tool for drug development and clinical trials.
Using a network of neurons grown on a chip, researchers can model disease pathways and test the effects of various drug compounds in a controlled, yet highly dynamic environment. This approach can significantly shorten the drug development timeline, as it allows for rapid iteration and refinement based on real-time biological feedback. Additionally, the ability to simulate human physiological responses more accurately than traditional in vitro methods can lead to more effective and personalized treatments, ultimately improving patient outcomes.
Transformative Impact on Drug Development
A) Accelerated Drug Discovery
Biological computing promises to significantly accelerate drug discovery by leveraging its massive parallelism to evaluate vast libraries of potential candidate compounds. DNA-encoded chemical libraries containing billions of small molecules can be rapidly screened in vitro for favourable interactions, enabling researchers to identify hit compounds much more efficiently than traditional high-throughput methods.
Pioneering experiments have already demonstrated DNA’s ability to discover novel antibiotic and antitumor compounds from random sequence pools. Moreover, molecular dynamics simulations powered by DNA computers could hasten structure-based drug design by predicting protein conformations at an unprecedented scale. This would facilitate in silico screening and the optimization of leads toward specific biomolecular targets.
Precision Medicine and Personalized Therapies
The most transformative application of biological computing lies in precision medicine and the development of personalized therapies. By integrating vast amounts of OMICs data through DNA storage and powerful algorithms, researchers can deeply characterize disease states and drug responses at the individual patient level. This enables the identification of subtle genomic variants and biomarkers that predict drug efficacy or toxicity for certain subpopulations.
For example, the simulation of cancer signalling networks through DNA logic has uncovered rare mutant kinases as targets for customized kinase inhibitors. Numerous targeted therapies have already emerged using this approach, including the BRAF inhibitors vemurafenib and dabrafenib for melanoma patients expressing the V600E mutation.
Another instance, the drug Kymriah, developed using gene editing and biological computing, has shown remarkable success in treating certain types of leukaemia by reprogramming a patient’s own T-cells to attack cancer cells.
Looking ahead, biological computers could simulate entire personalized models of human physiology to optimize treatment selection and dosing for each unique patient.
Clinical Trials and Testing
Simulation-based approaches also promise to revolutionize clinical trials using bio-computer hybrid systems. By culturing human tissues and organoids on microfluidic “body-on-a-chip” devices interfaced with supercomputers, researchers may soon model complete human biology virtually. This will enable unprecedented testing of investigational compounds without human subjects initially.
Computerized physiology platforms could help identify safe and effective doses more quickly while reducing expensive Phase I trials. Ultimately, the integration of biological and silicon-based computing heralds an era of “simulation medicine” that accelerates discovery and delivers more precise care than ever before.
Advances in Diagnostics and Disease Monitoring
Genomic Analysis
Genomic analysis has emerged as a pivotal tool in modern diagnostics, playing a crucial role in identifying genetic markers for a wide range of diseases. By examining an individual’s DNA, researchers can pinpoint specific genetic variations that predispose them to certain conditions, enabling early detection and more personalized treatment strategies. This approach has been particularly transformative in the field of oncology.
For instance, the identification of BRCA1 and BRCA2 gene mutations has significantly improved the early diagnosis and prevention strategies for breast and ovarian cancers. Similarly, advancements in genomic sequencing have facilitated the development of precision medicine, where treatments are tailored based on a patient’s unique genetic profile, thereby enhancing the effectiveness and reducing the side effects of therapies.
A notable case study is the implementation of the 100,000 Genomes Project by Genomics England, which aims to sequence 100,000 genomes to uncover new insights into rare diseases and cancers. This project has already led to the discovery of numerous genetic markers associated with various conditions, providing valuable data for the development of targeted therapies and improving diagnostic accuracy.
Real-Time Health Monitoring
The development of biosensors and advanced diagnostic tools is revolutionizing real-time health monitoring, offering unprecedented capabilities for continuous patient observation. These innovations have the potential to transform how diseases are tracked and managed, leading to more proactive and personalized healthcare.
Biosensors, which are analytical devices that combine a biological component with a physicochemical detector, can monitor a wide range of physiological parameters in real-time. For example, glucose sensors used by diabetic patients provide continuous monitoring of blood sugar levels, enabling timely interventions and better management of the condition. Beyond diabetes, biosensors are being developed to monitor cardiovascular health, detect infectious diseases, and even track cancer biomarkers in real time.
A groundbreaking development in this field is the creation of wearable and implantable diagnostic tools. These devices can continuously collect and transmit health data, providing healthcare professionals with real-time insights into a patient’s condition. For instance, the use of smartwatches equipped with ECG sensors allows for the continuous monitoring of heart rhythms, which can detect atrial fibrillation and other cardiac anomalies early. This continuous stream of data enables more accurate and timely diagnoses, potentially preventing severe health events and improving patient outcomes.
The potential for continuous patient monitoring extends beyond individual health management to broader public health applications. For example, during the COVID-19 pandemic, wearable biosensors were used to track symptoms and spread, providing critical data to inform public health responses and containment strategies.
Neuromorphic Computing in Pharma
Neuromorphic computing, also known as brain-inspired or brain-emulating computing, represents an emerging branch of biological computing focused specifically on mimicking the human brain’s cognitive abilities. Rather than computing via a traditional von Neumann architecture, neuromorphic systems are inspired by the neural architecture of the brain comprising vast numbers of simple but massively interconnected components. These systems leverage specialized hardware, such as memristors and spiking neural networks, to process information in a manner akin to biological neurons.
In the pharmaceutical industry, neuromorphic computing holds significant promise for advancing drug discovery, developing personalized treatments, and enhancing the understanding of complex biological systems.
Brain-Computer Interfaces
Neuromorphic computing is at the forefront of developing brain-computer interfaces (BCIs), which have transformative potential in the field of advanced prosthetics and neural implants. BCIs enable direct communication between the brain and external devices, allowing for the development of advanced prosthetics that can be controlled with neural signals. This technology offers life-changing benefits for individuals with limb loss or paralysis, providing them with greater mobility and independence.
Moreover, neuromorphic computing is playing a crucial role in addressing neurodegenerative diseases and mental health disorders. For example, neural implants powered by neuromorphic chips can simulate brain activity to restore lost functions in patients with conditions such as Parkinson’s disease and epilepsy. These implants can deliver precise electrical stimulation to affected brain areas, alleviating symptoms and improving quality of life. Additionally, BCIs are being explored for their potential in treating mental health conditions like depression and anxiety by modulating neural activity in real-time.
Cortical Labs’ Contributions
Cortical Labs is a pioneer in neuromorphic computing, making substantial contributions to its application in the pharmaceutical industry. One of their notable projects involves the development of “DishBrain,” a system that integrates human brain cells grown on microelectronic arrays. This hybrid platform combines the computational capabilities of biological neurons with the precision of silicon-based electronics, offering a powerful tool for drug testing and neurological research.
DishBrain’s ability to simulate complex neural networks has significant implications for pharma. It can model disease pathways more accurately than traditional in vitro methods, allowing researchers to study the effects of potential drug candidates on neural activity in real-time. This capability accelerates the drug development process by providing quicker and more reliable data on efficacy and safety. Furthermore, Cortical Labs’ technology enables the creation of personalized neural models, which can be used to develop tailored treatments for neurological disorders based on an individual’s unique neural profile.
Ethical and Technical Challenges
Ethical Considerations
The advent of advanced technologies in the pharmaceutical industry, particularly those involving genomic data and biological computing, brings forth significant ethical considerations. One of the foremost concerns is privacy. The collection and analysis of genomic data can reveal sensitive information about an individual’s predisposition to certain diseases, their ancestry, and even personal traits. Ensuring the confidentiality and security of this data is paramount to prevent misuse and discrimination. Cases of genetic data breaches have highlighted vulnerabilities in current systems, emphasizing the need for stringent data protection measures.
Moreover, the field of genetic engineering raises critical ethical questions. Techniques like CRISPR-Cas9 offer unprecedented capabilities to edit the human genome, which can be used to correct genetic disorders but also pose risks of unintended consequences and ethical dilemmas. For instance, the potential for “designer babies,” where genetic modifications are made for non-therapeutic enhancements, stirs significant ethical debates. Additionally, biosecurity concerns emerge with the possibility of creating genetically modified organisms that could have adverse environmental or health impacts if not properly controlled.
Technical Challenges
Beyond ethical considerations, there are substantial technical challenges associated with the scalability, reliability, and efficiency of biological computing systems. Biological computing, while promising, is still in its nascent stages and faces hurdles in scaling up for widespread application. The integration of biological components with traditional silicon-based systems requires overcoming issues related to compatibility and stability over time. For instance, maintaining the viability of biological elements like neurons or DNA within a computing framework poses significant technical difficulties.
Reliability is another critical issue. Biological systems are inherently variable, and ensuring consistent performance across different batches of biological components can be challenging. This variability can affect the accuracy and reproducibility of computational results, which is crucial in pharmaceutical applications where precision is paramount.
Efficiency also remains a key concern. While biological systems have the potential to operate at lower energy levels compared to conventional electronics, optimizing these systems to achieve the desired computational efficiency is an ongoing area of research. Achieving the right balance between speed, accuracy, and energy consumption requires innovative solutions and advancements in bio-computing technology.
Ongoing research is addressing these challenges through multidisciplinary approaches. For instance, efforts are being made to enhance the integration of biological and electronic components using advanced materials and fabrication techniques. Researchers are also developing methods to improve the stability and longevity of biological elements within computing systems. Additionally, advancements in synthetic biology are paving the way for more reliable and efficient bio-computing platforms.
Standardization of parts and platforms will further aid progress. The international Genetically Engineered Machine (iGEM) competition exemplifies efforts towards building a standardized “biology toolkit” (iGEM, 2022). Continued investment in multidisciplinary research linking computer science, engineering, and synthetic biology will also be imperative to overcoming present limitations. With careful focus on responsible innovation and humanistic values like beneficence, biological computing holds enormous potential to revolutionize medicine if its full technical and ethical issues are addressed proactively through open collaboration on a global scale. The desire to optimize human wellness through pioneering technology must be balanced with safeguarding human dignity.
Future Prospects and Industry Trends
Emerging Technologies
The future for biological computing is tremendously promising as both the technology and its applications continue to evolve rapidly. On the technological front, the new field of quantum biology exploring quantum effects in living systems holds particular interdisciplinary promise. Researchers are investigating quantum coherence, entanglement and tunnelling phenomena observed in photosynthesis, olfaction and other natural processes that could enable new modes of ultra-fast, hyper-parallel biological information processing. Other emerging areas like synthetic quantum chemical circuits, DNA and molecular spintronics may synergize quantum effects with biological platforms in the years ahead.
A fascinating aspect of quantum biology is its potential to enable more precise simulations of molecular interactions, which is crucial for drug discovery and development. For instance, quantum computing could simulate complex biomolecular structures and their interactions with potential drug candidates at a level of detail that is currently unattainable, thereby accelerating the drug discovery process and improving the accuracy of predicting therapeutic outcomes.
Future advancements to watch in this space include the development of hybrid quantum-classical algorithms specifically tailored for biological applications. These algorithms could leverage the strengths of both quantum and classical systems to solve complex biological problems more efficiently. Additionally, researchers are exploring the use of quantum sensors to detect and analyse biological phenomena with unprecedented sensitivity and precision, which could revolutionize diagnostics and personalized medicine.
Industry Adoption and Investment
The pharmaceutical industry is increasingly recognizing the transformative potential of biological computing, leading to significant investments and strategic initiatives. Major pharmaceutical companies are allocating substantial resources to explore and develop biological computing technologies, aiming to enhance their R&D capabilities and gain a competitive edge.
For example, Pfizer has invested in partnerships with tech companies specializing in bio-computing to integrate advanced computational methods into their drug discovery pipelines. Recently, Pfizer announced a collaboration with the biotechnology company Ginkgo Bioworks to harness synthetic biology and bio-computing for the development of new therapeutics. Similarly, Johnson & Johnson has launched initiatives to incorporate biological computing into their R&D processes, focusing on improving the efficiency and accuracy of drug development.
Industry trends indicate a growing emphasis on personalized medicine and the use of big data analytics, both of which are well-supported by advances in biological computing. The ability to analyze vast amounts of genomic and proteomic data quickly and accurately allows for the development of highly personalized treatment regimens, tailored to the genetic profiles of individual patients. This trend is evident in the increasing number of precision medicine initiatives and the integration of bio-computing technologies in clinical trials to enhance data collection and analysis.
Furthermore, venture capital investments in biotech startups focusing on bio-computing have surged, reflecting the industry’s confidence in the potential of these technologies. According to a report by CB Insights, investment in biotech startups leveraging biological computing and synthetic biology reached a record high of $19 billion in 2023, highlighting the growing interest and financial commitment to this field.
Conclusion
Biological computing has made immense progress since its inception and is poised to transform the pharmaceutical industry through its innovative applications spanning drug discovery, personalized medicine, and health diagnostics. By harnessing the massive parallelism and molecular recognition abilities of biological processes, it offers unprecedented opportunities to characterize disease mechanisms, screen compounds, and model human physiology at an exponential scale compared to conventional approaches. Though still in its relative infancy, the rapid developments in DNA sequencing, synthetic biology, organ-on-chip engineering, and quantum effects applied to living systems suggest its capacity to replicate the most sophisticated wetware may not be too far off. This could revolutionize our understanding and treatment of disorders previously considered incurable.
As biological computers merge more seamlessly with AI, cloud computing, electronics and other nascent technologies, their impact will become all the more stratospheric. Yet, realizing biological computing’s full promise to advance human health also requires proactively addressing issues of data privacy, biosafety, access disparity and responsible innovation through inclusive public dialogue and wise governance frameworks.
As the boundary between engineering and biology inevitably blurs in the years ahead, upholding principles like transparency, consent and fairness will grow ever more important to earning public trust. Ultimately, if harnessed judiciously as a force multiplier for the greater good, biological computing’s convergence with medicine holds tremendous hope for optimizing humanity’s well-being in this new age of Synthetic Biology. Its dawn heralds one of the most innovative and thoughtful eras in the history of science and its relationship with society.
Found this article interesting?
P.S. Here are 5 ways we can help you accelerate your Pharma AI results:
1. Follow Dr Andrée Bates LinkedIn Profile Now
Dr Bates posts regularly about AI in Pharma so if you follow her you will get even more insights.
2. Listen to our AI for Pharma Growth Podcast
Revolutionize your team’s AI solution vendor choice process and unlock unparalleled efficiency and save millions on poor AI vendor choices that are not meeting your needs! Stop wasting precious time sifting through countless vendors and gain instant access to a curated list of top-tier companies, expertly vetted by leading pharma AI experts.
Every year, we rigorously interview thousands of AI companies that tackle pharma challenges head-on. Our comprehensive evaluations cover whether the solution delivers what is needed, their client results, their AI sophistication, cost-benefit ratio, demos, and more. We provide an exclusive, dynamic database, updated weekly, brimming with the best AI vendors for every business unit and challenge. Plus, our cutting-edge AI technology makes searching it by business unit, challenge, vendors or demo videos and information a breeze.
Discover vendors delivering out-of-the-box AI solutions tailored to your needs.
Identify the best of the best effortlessly.
Anticipate results with confidence.
Transform your AI strategy with our expertly curated vendors that walk the talk, and stay ahead in the fast-paced world of pharma AI!
Get on the wait list to access this today. Click here.
4. Take our FREE AI for Pharma Assessment
This assessment will score your current leveraging of AI against industry best practice benchmarks, and you’ll receive a report outlining what 4 key areas you can improve on to be successful in transforming your organization or business unit.
Plus receive a free link to our webinar ‘AI in Pharma: Don’t be Left Behind’. Link to assessment here
5. Learn more about AI in Pharma in your own time
We have created an in-depth on-demand training about AI specifically for pharma that translate it into easy understanding of AI and how to apply it in all the different pharma business units — Click here to find out more.